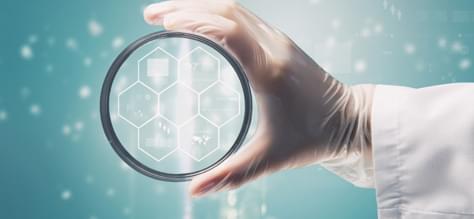
Enzymes
Historically, biological catalysis has been used by mankind for thousands of years, ever since fermentation was discovered as a process for brewing and bread-making in ancient Egypt. It was not until the 19th century AD, however, that scientists addressed the question of whether the entity responsible for processes such as fermentation was a living species or a chemical substance. In 1897 Eduard Buchner published the observation that cell-free extracts of yeast containing no living cells were able to carry out the fermentation of sugar to alcohol and carbon dioxide. He proposed that a species called ‘zymase’ found in yeast cells was responsible for fermentation. The biochemical pathway involved in fermentation was subsequently elucidated by Embden and Meyerhof – the first pathway to be discovered.
The exquisite selectivity of enzyme catalysis was recognized as early as 1894 by Emil Fischer, who demonstrated that the enzyme which hydrolyses sucrose, which he called ‘invertin’, acts only upon a-d-glucosides, whereas a second enzyme ‘emulsin’ acts only upon b-d-glucosides. He deduced that these two enzymes must consist of ‘asymmetrically built molecules’, and that ‘the enzyme and glucoside must fit each other like a lock and key to be able to exert a chemical influence upon each other’. Fischer’s ‘lock and key’ hypothesis remained a powerful metaphor of enzyme action for many years. The crystallization in 1926 of the enzyme urease from Jack beans by Sumner proved beyond doubt that biological catalysis was carried out by a chemical substance.
The recognition that biological catalysis is mediated by enzymes heralded the growth of biochemistry as a subject, and the elucidation of the metabolic pathways catalyzed by enzymes. Each reaction taking place on a biochemical pathway is catalyzed by a specific enzyme. Without enzyme catalysis the uncatalyzed chemical process would be too slow to sustain life. Enzymes catalyze reactions involved in all facets of cellular life: metabolism (the production of cellular building blocks and energy from food sources); biosynthesis (how cells make new molecules); detoxification (the breakdown of toxic foreign chemicals); and information storage (the processing of DNA).
In any given cell there are present several thousand different enzymes, each catalyzing its specific reaction. How does a cell know when it needs a particular enzyme? The production of enzymes is controlled by a cell’s DNA, both in terms of the specific structure of a particular enzyme and the amount that is produced. Thus different cells in the same organism have the ability to produce different types of enzymes and to produce them in differing amounts according to the cell’s requirements.
Since the majority of the biochemical reactions involved in cellular life are common to all organisms, a given enzyme will usually be found in many or all organisms, albeit in different forms and amounts. By looking closely at the structures of enzymes from different organisms which catalyze the same reaction, we can in many cases see similarities between them. These similarities are due to the evolution and differentiation of species by natural selection. So by examining closely the similarities and differences of an enzyme from different species we can trace the course of molecular evolution, as well as learning about the structure and function of the enzyme itself.
Recent developments in biochemistry, molecular biology and X-ray crystallography now allow a far more detailed insight into how enzymes work at a molecular level. We now have the ability to determine the amino acid sequence of enzymes with relative ease, whilst the technology for solving the 3D structure of enzymes is developing apace. We also have the ability to analyze their 3D structures using molecular modelling and then to change the enzyme structure rationally using site-directed mutagenesis. We are now starting to enter the realms of enzyme engineering where, by rational design, we can modify the genes encoding specific enzymes, creating the ‘designer genes’ of the title. These modified enzymes could in future perhaps be used to catalyze new types of chemical reactions, or via gene therapy used to correct genetic defects in cellular enzymes which would otherwise lead to human diseases.
Coenzymes
At the same time as the discovery of enzymes in the late 19th and early 20th centuries, a class of biologically important small molecules was being discovered which had remarkable properties to cure certain dietary disorders. These molecules were christened the vitamins, a corruption of the phrase ‘vital amines’ used to describe their dietary importance (several of the first-discovered vitamins were amines, but this is not true of all the vitamins). The vitamins were later found to have very important cellular roles, shown in Table 1.
Table 1. The vitamins.
Vitamin | Chemical name | Deficiency disease | Biochemical function | Coenzyme chemistry |
A | Retinol | Night blindness | Visual pigments | — |
B1 | Thiamine | Beriberi | Coenzyme (TPP) | Decarboxylation of a-keto acids |
B2 | RiboXavin | Skin lesions | Coenzyme (FAD, FMN) | 1e–=2e– redox chemistry |
Niacin | Nicotinamide | Pellagra | Coenzyme (NAD) | Redox chemistry |
B6 | Pyridoxal | Convulsions | Coenzyme (PLP) | Reactions of a-amino acids |
B12 | Cobalamine | Pernicious anaemia | Coenzyme | Radical re-arrangements |
C | Ascorbic acid | Scurvy | Coenzyme, anti-oxidant | Redox agent (collagen formation) |
D | Calciferols | Rickets | Calcium homeostasis | — |
E | Tocopherols | Newborn haemolytic anaemia | Anti-oxidant | — |
H | Biotin | Skin lesions | Coenzyme | Carboxylation |
K | Phylloquinone | Bleeding disorders | Coenzyme, anti-oxidant | Carboxylation of glutamyl peptides |
Folic acid | Megaloblastic anaemia | Coenzyme (tetrahydrofolate) | 1-carbon transfers | |
Pantothenic acid | Burning foot syndrome | Coenzyme (CoA, phosphopantotheine) | Acyl transfer |
The first demonstration of the importance of vitamins in the human diet took place in 1753. A Scottish naval physician, James Lind, found that the disease scurvy, prevalent amongst mariners at that time, could be avoided by deliberately including green vegetables or citrus fruits in the sailors’ diets. This discovery was used by Captain James Cook to maintain the good health of his crew during his voyages of exploration in 1768–76. The active ingredient was elucidated much later as vitamin C, ascorbic acid.
The first vitamin to be identified as a chemical substance was thiamine, lack of which causes the limb paralysis beriberi. This nutritional deficiency was first identified in the Japanese Navy in the late 19th century. The incidence of beriberi in sailors was connected with their diet of polished rice by Admiral Takaki, who eliminated the ailment in 1885 by improvement of the sailors’ diet. Subsequent investigations by Eijkman identified a substance present in rice husks able to cure beriberi. This vitamin was subsequently shown to be an essential ‘cofactor’ in cellular decarboxylation reactions.
Over a number of years the family of vitamins shown in Table 1.1 was identified and their chemical structures elucidated. Some, like vitamin C, have simple structures, whilst others, like vitamin B12, have very complex structures. It has taken much longer to elucidate the molecular details of their biochemical mode of action. Many of the vitamins are in fact coenzymes: small organic cofactors which are used by certain types of enzyme in order to carry out particular classes of reaction.
Reference:
1. Bugg T D H. Introduction to Enzyme and Coenzyme Chemistry. Natural Product Reports, 2005, 22(1):127-127.
Related Products at Creative Enzymes:
Related Service at Creative Enzymes:
Leave a Reply